Heavy metal contamination of water through the discharge of industrial wastewater is a growing environmental issue worldwide. The most common heavy metal contaminating industries are metal plating, mining operations, battery manufacturing, paints and pigments, ammunition, ceramic and glass industries. The wastewater from these industries commonly contain toxic heavy metals like Cd (II), Pb (II), Cu (II), Ni (II), and Cr (VI) [1]. Industrialization moves so rapid that the discharge of heavy metals into the environment increases accordingly [2]. Numbers of treatment methods like ion exchange [3], precipitation, filtration, oxidation-reduction, membrane separation, and adsorption [3], [4] are known for separation and removal of metallic species from aqueous solutions and wastewaters. It has been reported that application of these methods is limited due to high operational costs or insufficient removal efficiencies to meet the environmental requirements. The most familiar metals refer to heavy metals having density more than or equal to 6.0 g/mL such as:
Cadmium (8.65 g/mL);
Chromium (7.19 g/mL);
Cobalt (8.90 g/mL);
Copper (8.95 g/mL);
Lead (11.34 g/mL);
Mercury (13.53 g/mL);
Nickel (8.91 g/mL);
Zinc (7.14 g/mL).
The hostile effects of cadmium on human lives include high blood pressure, kidney damage, destruction of testicular tissues and red blood cells [5]. Chromium occurs in two stable oxidation states, Cr (III) and Cr (VI), in nature. The Cr (III) is relatively innocuous, but Cr (VI) is toxic, carcinogenic and mutagenic in nature, and highly mobile in soil and aquatic system [6].
Several investigations have been made in this area utilizing number of materials like:
Sunflower stalks [7];
Almond-shell carbon [8];
Sugarcane baggase [9];
Oak saw dust [10];
Wheat bran [11];
Maize bran [12], etc.
Varanasi is a city of temples in India and more than 1,000,000 pilgrims visit the city each year. Marigold flowers are very cheap and easily available around the year. Most of the poor people generally use the garlands of marigold flowers to pray and bow their heads to Lord Shiva. Hence, a lot of flower waste is generated daily. Present study deals with series of batch adsorption experiments to assess the potentiality of dried marigold flower for removal of Cr (VI) and Cd (II) from aqueous solutions. The adsorption kinetics, isotherm models and thermodynamic parameters were determined to understand the mechanism and nature of adsorption process.
The marigold flower wastes were collected from Lord Shiva temple, Varanasi in the University campus. It was washed with sufficient amount of distilled water to remove water soluble substances and surface adhered impurities. The washed marigold flower wastes were then dried at 100 °C in hot air oven for 12 h, then ground with the help of grinder and stored in air tight plastic containers.
All chemicals and reagents used were of the analytical reagent grade and obtained from E. Merck, India. The pH of the solution was measured by using pH meter (LI 120, Elico, India). A shaking speed incubator (Model NSW-133, Calton, and New Delhi, India) was used for shaking the content to execute batch adsorption experiments. An oven (S.M. Scientific Instruments Pvt. Ltd., New Delhi) was used for drying the adsorbent. To examine the mineral identification of adsorbent, X-Ray Diffraction (XRD) studies were done. The XRD data was obtained by 18 kW rotating anode based powder diffractometer (Rigaku, Japan) fitted with a curved crystal monochromator in the diffracted beam operating at 40 kV and 150 mA. Nature of bonding was visualized by FTIR JASCO spectrophotometer (Model-5300). The particle size of the adsorbent was determined by sieve analysis. The concentration of Cd (II) and Cr (VI) before and after adsorption was measured using a UV-Visible spectrophotometer (SL 159, Elico, India).
The stock solution of Cd (II) (1,000 mg/L) and Cr (VI) (1,000 mg/L) were prepared by dissolving AR grade Cd (Ν03)2·4Η20 and K2Cr2O7 in deionized water. The stock solutions were further diluted with deionized water to get desired concentration of Cd (II) and Cr (VI) for experimentations.
Adsorption experiments were conducted in a series of glass stoppered conical flasks by agitating a weighed amount (0.8 g for Cr (VI) and 0.2 g for Cd (II)) of adsorbent with 100 mL aqueous solution of Cr (VI) or Cd (II). The solution pH was adjusted to the desired value by adding 0.1 M HCl or 0.1 M NaOH. The flasks were shaken at 30 °C using a mechanical shaker for a desirable time. At the end of pre-determined time intervals the flasks were withdrawn from shaker and supernatant liquid after filtering the contents was analysed spectrophotometrically to find out remaining heavy metal ion concentration. The percent removal of Cd (II) or Cr (VI) was calculated for each run by following eq. (1):
Adsorption capacities of the dried marigold flower for Cd (II) or Cr (VI) were calculated using the equation as given below:
where:
qe is adsorption capacity for heavy metal removal per unit mass of adsorbent [mg/g];
M is mass of adsorbent [g];
V is volume of sample [L];
C0 is the initial concentration of heavy metal in the test solution [mg/L];
Ct is concentration at any time t [mg/L];
and Ce is the final equilibrium concentration of heavy metal in solution [mg/L].
All the experiments were accomplished in duplicate with uncertainty of ± 0.1% for the measured data, and the average values were reported.
To understand the mechanism of adsorption of heavy metal onto adsorbent, it is necessary to determine point of zero charge of the adsorbent (pHzpc). The specific adsorption of cation shifts pHzpc towards lower value, whereas the specific adsorption of anions shifts pHzpc towards higher value. The pHzpc of dried marigold flower was found as 4.12. XRD study of dried marigold flower was performed by powder diffractometer (Rigaku, Japan) fitted with a curved crystal monochromator in the diffracted beam operating at 40 kV and 150 mA. The data were collected in the angular (2ϴ) range from 20 to 120 degrees with a step size of 0.02 degrees at a rate of 2 degrees per minute. The recorded XRD profiles were compared with standard profiles by the help of JCPDF files and the compounds such as SiO2, MgO, CaO and Al2O3 were identified in the sample. Dried marigold flower was found to contain carbon and silica based materials that bound the heavy metal ions from aqueous solution.
The functional group is one of the keys to understand the mechanism of heavy metal binding on dried marigold flower. Fourier Transform Infrared Spectroscopy (FTIR) of adsorbent for identifying the functional groups in 4,000-400 cm−1 range was shown in Figure 1. The strong absorption band at 3,986-3,710 cm−1 was stretching vibrations of surface hydroxyl groups. The band 2,963 cm−1 was assigned to symmetric vibration of C-H group especially alkenes. The strong bands at 2,348 and 2,345 cm−1 were stretching vibrations of N-H and C=O groups probably due to amines and ketones. The bands at 1,723 and 1,728 cm−1 were, respectively, attributed to carbonyl groups (mainly aldehydes and ketones) conjugated not to aromatic rings. The bands at 1,029 and 1,034 cm−1 are stretching vibration of C-O-C and O-H of polysaccharides. The bands at 1,526 and 1,528 cm−1 were an identification of COO and C=O groups. The N-H stretching appears at 3,437 cm−1. The peaks at 631, 604 and 527 cm−1 were assigned for bending modes of aromatic compounds. FTIR spectrum of dried marigold flower showed that it was composed of polymeric OH group, C-H and COO groups of polysaccharides. All these groups were known to have strong affinity of heavy metal adsorption.
FTIR plot of dried marigold flower
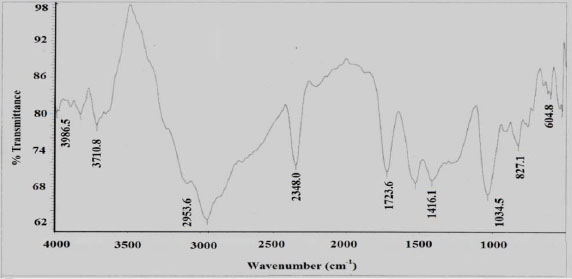
Figure 2 showed the effect of contact time on batch adsorption of Cd (II) and Cr (VI) at 30 °C. Initially, the percent removal increased slowly with time and then it reached a maximum value in approximately 75 min for Cd (II) and 105 min for Cr (VI), respectively. After that it decreased gradually to reach the equilibrium value. The maximum removal of Cd (II) and Cr (VI) was found as 78 and 92%, respectively at initial concentration of 10 mg/L. The atomic radius of Cr (VI) (1.28 Å) is less than that of Cd (II) (1.51 Å). Higher the atomic radius of metal, the lower is its diffusive mobility in an aqueous medium. Therefore, the diffusive mobility of Cd (II) from bulk of the liquid to the surface of adsorbent is slower as compared to Cr (VI) in aqueous solutions which may lead to the lower adsorption of Cd (II) than Cr (VI) by the adsorbent. For both Cd (II) and Cr (VI), the percent removal decreases with increase in initial concentration of heavy metal ions. The variation in percent removal of Cd (II) and Cr (VI) ions may be due to the fact that initially all sites on the surface of adsorbent were empty and the solute concentration gradient was relatively high for increased initial metal ion concentration [13]. Consequently in the latter stage percent removal decreased with increase in time, depending on the less number of vacant sites available which may be difficult to occupy due to repulsive forces between solute molecules of the adsorbent surface and bulk of the liquid phase [14].
Effect of contact time on removal of Cd (II) and Cr (VI) ions
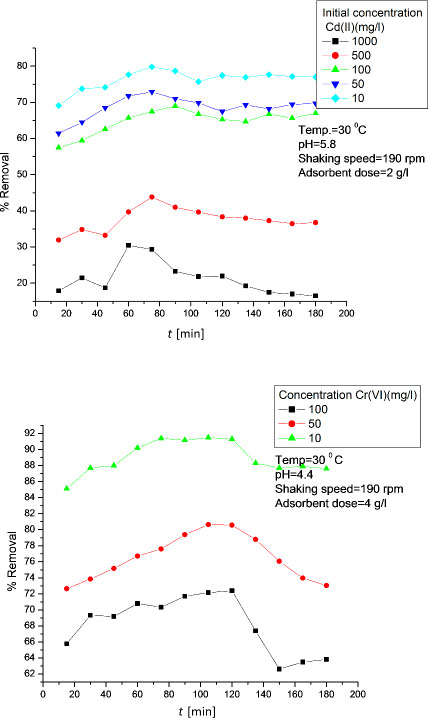
To achieve maximum percent removal of Cd (II) and Cr (VI) ions, adsorbent dose was varied from 0.5 to 10 mg/L for Cd (II) and 2 to 10 mg/L for Cr (VI). It was noticed that adsorbent doses of 2 g/L and 8 g/L were enough for maximum removal of Cd (II) and Cr (VI) ions, respectively (Figure 3). At initial stage, increase in percent removal of both heavy metal ions with increase in adsorbent dose was due to the availability of more and more adsorbent surfaces for adsorption. It was also seen from Figure 3 that a further increase in adsorbent dose (beyond 8 g/L) did not affect the adsorption greatly for Cr (VI) ions but for Cd (II) ions the decrease in adsorption after maximum removal with increase in adsorbent dose was due to the adsorption sites remained unsaturated during the adsorption reaction. There may be the larger resistance for Cd (II) ions to penetrate from surface to the interior of the adsorbent indicating the decrease in adsorption later on with adsorbent dose [15].
Effect of adsorbent dose on removal of Cd (II) and Cr (VI) ions
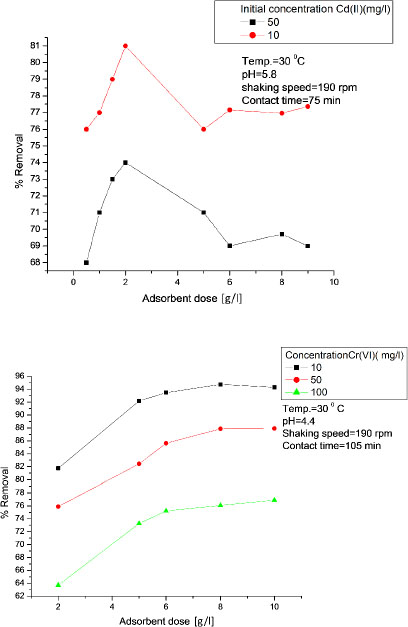
The pH is an important parameter which affects metal chemistry depending on surface charge of the heavy metal binding sites onto the adsorbent [14]. To see the effect of pH on removal of Cd (II) and Cr (VI), the initial pH was changed from 1 to 9 and the results are shown in Figure 4. It was observed that percent removal increases as the initial pH was increased and attained a maximum value at a particular pH. After that, it was found to decrease in both Cd (II) and Cr (VI) adsorption. The maximum removal of Cd (II) and Cr (VI) were achieved at pH 3 and 5, respectively. The decrease of percent removal at higher pH may be attributed to the hydroxide formation of Cd (II) and Cr (VI) [16].
Effect of pH on percent removal of Cd (II) and Cr (VI) ions
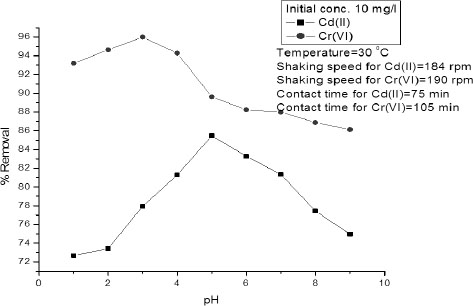
The effect of temperature on percent removal of Cd (II) and Cr (VI) was investigated over the temperature range of 30 to 50 °C. The results were shown in Figure 5. It was evident from Figure 5 that the maximum adsorption was observed at 30 °C for both Cd (II) and Cr (VI) ions and it was decreased with increasing temperature. The increase in adsorption with decreasing temperature showed exothermic nature of adsorption process [13].
Effect of temperature on percent removal of Cd (II) and Cr (VI) ions
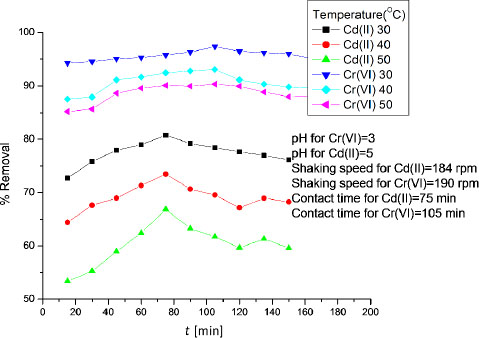
In this study, equilibrium data were analyzed by employing well known Langmuir and Freundlich adsorption isotherms [16] which are as follows, respectively:
where:
qe is amount adsorbed [mg/g];
Ce is the equilibrium concentration of the adsorbate [mg/L];
Q0 and b are the Langmuir constants related to maximum adsorption capacity and energy of adsorption, respectively;
KF and n are Freundlich constants related to adsorption capacity and adsorption intensity, respectively.
The regression values of all isotherms constants were given in Table 1. Freundlich isotherm for adsorption of Cd (II) and Cr (VI) was fitted better than the Langmuir isotherm, which is evident from value of regression coefficient (R2).
Langmuir and Freundlich constants for adsorption of Cd (II) and Cr (VI)
Adsorbates |
Langmuir parameters |
Freundlich parameters |
||||
---|---|---|---|---|---|---|
Qo [mg/g] |
b [l/mg] |
R2 |
KF |
n |
R2 |
|
Cd (II) |
55.56 |
0.014 |
0.968 |
1.33 |
1.420 |
0.978 |
Cr (VI) |
11.11 |
0.140 |
0.989 |
1.11 |
1.686 |
0.999 |
The prediction of adsorption kinetics is most important factor as it describes the solute uptake rate which intern controls the residence time of solute uptake at the solid liquid interface [17], [18]. In order to determine the adsorption kinetics for Cd (II) and Cr (VI) removal, Lagergren kinetic model was tested. The pseudo first order model proposed by Lagergren can be expressed as [7]:
where:
qe is the amount of adsorbed [mg/g] at equilibrium;
qt is the amount adsorbed [mg/g] at time t;
and k1 the adsorbent rate constant [min−1].
The values of k1 were given in Table 2. The simplified form of pseudo second order model can be written as [14]:
where k2 is the adsorption rate constant (g/mg.min). The values of k2 were given in Table 2. The values of correlation coefficient (R2) confirmed the better applicability of pseudo second- order model as compared to pseudo first order rate equation for the adsorption of Cd (II) and Cr (VI). The intraparticle diffusion varies with square root of time is also attempted using the following expression [14]:
where kid (mg/g min1/2) is the rate constant of intraparticle diffusion. The values of kid were given in Table 2. The Cd (II) and Cr (VI) adsorption onto dried marigold flower indicated that some degree of boundary layer may also control the adsorption process and intraparticle diffusion may not be the sole rate- limiting step.
Kinetic parameters of Cd (II) and Cr (VI) adsorption onto waste marigold
Adsorbates |
Pseudo first order model |
Pseudo second order model |
||||
---|---|---|---|---|---|---|
k1 |
qe |
R2 |
k2 |
qe |
R2 |
|
Cd (II) |
0.032 |
2.630 |
0.893 |
0.104 |
3.906 |
0.994 |
Cr (VI) |
0.051 |
4.315 |
0.756 |
0.683 |
1.099 |
0.998 |
Thermodynamic parameters viz. Gibbs free energy (ΔG°), the enthalpy (ΔΗ°) and the entropy (ΔS°) for the adsorption process were obtained using the following eqs. (9 - 11):
where:
R is the ideal gas constant [kJ/mol K];
T is temperature in K;
and b is the Langmuir constant.
The values of ΔG°, ΔΗ°, ΔS° were reported in Table 3. Negative value of ΔG° indicated spontaneous nature of the process for removal of Cd (II) and Cr (VI) ions by dried marigold flower. Negative values of ΔΗ° suggested exothermic nature of the process. Free energy increased with increase in temperature indicating a decrease in adsorption at higher temperature which also confirmed the exothermic nature of adsorption process (Table 3). The negative values of ΔS° indicated the stability of adsorbed species during adsorption process.
Thermodynamic parameters for Cd (II) and Cr (VI) adsorption onto waste marigold
Adsorbates |
ΔG° [kJ/mol] |
ΔH° [kJ/mol] |
ΔS° [kJ/mol K] |
||
---|---|---|---|---|---|
30 °C |
40 °C |
50 °C |
|||
Cd (II) |
-10.119 |
-10.053 |
-9.904 |
-12.994 |
-0.009 |
Cr (VI) |
-6.066 |
-5.912 |
-5.682 |
-11.523 |
-0.018 |
In order to test the reusability of heavy metal loaded adsorbent, it was subjected to successive adsorption-desorption cycles. This procedure was tried three times with 100 mL of 0.1 M HCl or 0.1 M NaOH as eluted solution. The percentage adsorption or desorption values were depicted in Table 4 and the results were very promising and showed that 0.1 M HCl solution is suitable eluent for Cd (II) and 0.1 M NaOH is suitable eluent for Cr (VI) ions. There was only a slight decrease of heavy metal adsorbed (5.2% for Cd (II) and 5.5% for Cr (VI)), desorbed (4.8% for Cd (II) and 5.4% for Cr (VI)) and a small loss of mass adsorbent (10.2% for Cd (II) and 9.8% for Cr (VI)). These results showed the ability of dried marigold flower to efficiently adsorb / desorb both Cd (II) and Cr (VI) ions.
Adsorption-desorption cycles of waste marigold for Cd (II) and Cr (VI) ions
Adsorbates |
Cycles |
0.1 M HCl solution |
0.1 M NaOH solution |
||
---|---|---|---|---|---|
Adsorption [%] |
Desorption [%] |
Adsorption [%] |
Desorption [%] |
||
Cd (II) |
1 |
83.1 |
83.9 |
83.1 |
63.1 |
2 |
79.8 |
81.6 |
54.3 |
52.5 |
|
3 |
77.9 |
79.1 |
46.8 |
41.8 |
|
Cr (VI) |
1 |
95.8 |
46.5 |
95.8 |
96.7 |
2 |
41.7 |
39.3 |
93.4 |
94.2 |
|
3 |
28.6 |
30.4 |
90.3 |
91.3 |
Waste marigold flower was found to be an efficient adsorbent for removal of Cd (II) and Cr (VI) from aqueous solutions. The pHzpc of the adsorbent was found as 4.12. The adsorption of Cd (II) and Cr (VI) followed pseudo second order rate expression with boundary layer control as one of the rate determining step. The adsorption isotherm data of Cd (II) and Cr (VI) fitted well the Freundlich isotherm. The 0.1 M HCl and 0.1 M NaOH solution was shown appropriate eluents for Cd (II) and Cr (VI), respectively during desorption operation. The thermodynamic parameters confirmed the exothermic nature of adsorption of both Cd (II) and Cr (VI) from aqueous solutions.
A New Approach to Modification of Natural Adsorbent for Heavy Metal Adsorption ,Bioresour. Technol. , Vol. Vol. 99 , :2516-25272008, https://doi.org/10.1016/j.biortech.2007.04.037
, Study on the Preparation of Orange Peel Cellulose Adsorbents and Biosorption of Cd (II) from Aqueous Solution ,Sep. Purif. Technol. , Vol. Vol. 55 , :69-752007, https://doi.org/10.1016/j.seppur.2006.10.025
, Efficient Facilitated Transport of Lead, Cadmium, Zinc and Silver across a Flat Sheet-supported Liquid Membrane Mediated by a Lasalocid A ,Sep. Sci. Technol. , Vol. Vol, 37 , :1851-18602002, https://doi.org/10.1081/SS-120003047
, Modeling Cd Adsorption in Single and Binary Adsorbent (PAC) System ,J. Environ. Eng. Div. ASCE , Vol. Vol. 104 , :863-8781978
, Removal of Cadmium (II) from Aqueous Solutions by Adsorption on Agricultural Waste Biomass ,J. Hazard. Mater. , Vol. Vol. 154 , :1149-11572008, https://doi.org/10.1016/j.jhazmat.2007.11.040
, Biosorption of Hexavalent and Trivalent Chromium by Palm Flower (Borassus aethiopum) ,Chem. Eng. J. , Vol. Vol. 141 , :99-1112008, https://doi.org/10.1016/j.cej.2007.10.026
, Sunflower stalks as Adsorbents for the Removal of Metal Ions from Waste Water ,Ind. Eng. Chem. Res. , Vol. Vol. 37 , :1324-13281998, https://doi.org/10.1021/ie970468j
, Copper Ion Removal by Almond Shell Carbons and Commercial Carbons: Batch and Column Studies ,Sep. Sci. Technol. , Vol. Vol. 37 , :2369-2382002, https://doi.org/10.1081/ss-120003518
, Lignocellulosic Materials as Potential Biosorbents of Trace Toxic Metals from Wastewater ,Ind. Eng. Chem. Res. , Vol. Vol. 41 , :3580-35852002, https://doi.org/10.1021/ie020023h
, Modified Hardwood Saw Dust as Adsorbent of Heavy Metal Ions from Water ,Wood Sci. Technol. , Vol. Vol. 40 , :217-2272006, https://doi.org/10.1007/s00226-005-0061-6
, Adsorption Characteristics of Wheat Bran towards Heavy Metal Cations ,Sep. Sci. Technol. , Vol. Vol. 38 , :197-2072004, https://doi.org/10.1016/j.seppur.2003.11.005
, Adsorption, Kinetics and Equilibrium Studies on Removal of Cr (VI) from Aqueous Solutions using different Low-cost Adsorbents ,Chem. Eng. J. , Vol. Vol. 137 , :529-5412008
, Mass Transfer and Related Phenomena for Cr(VI) Adsorption from Aqueous Solutions onto Mangifera Indica Sawdust ,Chem. Eng. J. , Vol. Vol. 218 , :138-1462013, https://doi.org/10.1016/j.cej.2012.12.054
, Removal of Pb (II) from Aqueous Solution by Adsorption using Activated Tea Waste ,Korean J. Chem. Eng. , Vol. Vol. 27 , :144-1512010, https://doi.org/10.1007/s11814-009-0304-6
, Optimization of Parameters for Adsorption of Metal Ions onto Rice Husk Ash using Taguchi's Experimental Design Methodology ,J. Chem. Eng. , Vol. Vol. 140 , :136-1442008, https://doi.org/10.1016/j.cej.2007.09.030
, Kinetics, Thermodynamic and Equilibrium Study of Cr (VI) Adsorption from Aqueous Solutions by NCL Coal Dust ,Korean J. Chem. Eng. , Vol. Vol. 29 , :1782-17872012, https://doi.org/10.1007/s11814-012-0092-2
, Heavy Metal Removal by Olive Pomace: Biosorbent Characterisation and Equilibrium Modeling ,Chem. Eng. Sci. , Vol. Vol. 58 , :4709-47172003, https://doi.org/10.1016/j.ces.2003.08.001
, Removal of Pb (II) Ions from Aqueous Solution using activated Tea Waste: Adsorption on a Fixed- bed Column ,J. Environ. Manage. , Vol. Vol. 90 , :3266-32712009, https://doi.org/10.1016/j.jenvman.2009.05.025
,